A research team, affiliated with UNIST has developed a novel synthetic approach that could dramatically improve the durability of silicon (Si)-based anode materials that are regarded as high capacity anodes for next generation lithium ion batteries (LIBs). According to the research team, the newly-synthesized anode materials not only have a smaller particle size, but also exhibit an excellent cycle life.
This breakthrough has been carried out by Distinguished Professor Jaephil Cho and his research team in the School of Energy and Chemical Engineering at UNIST. Published in the December 2021 issue of Nature Energy, their findings have been featured on the cover of the print edition.
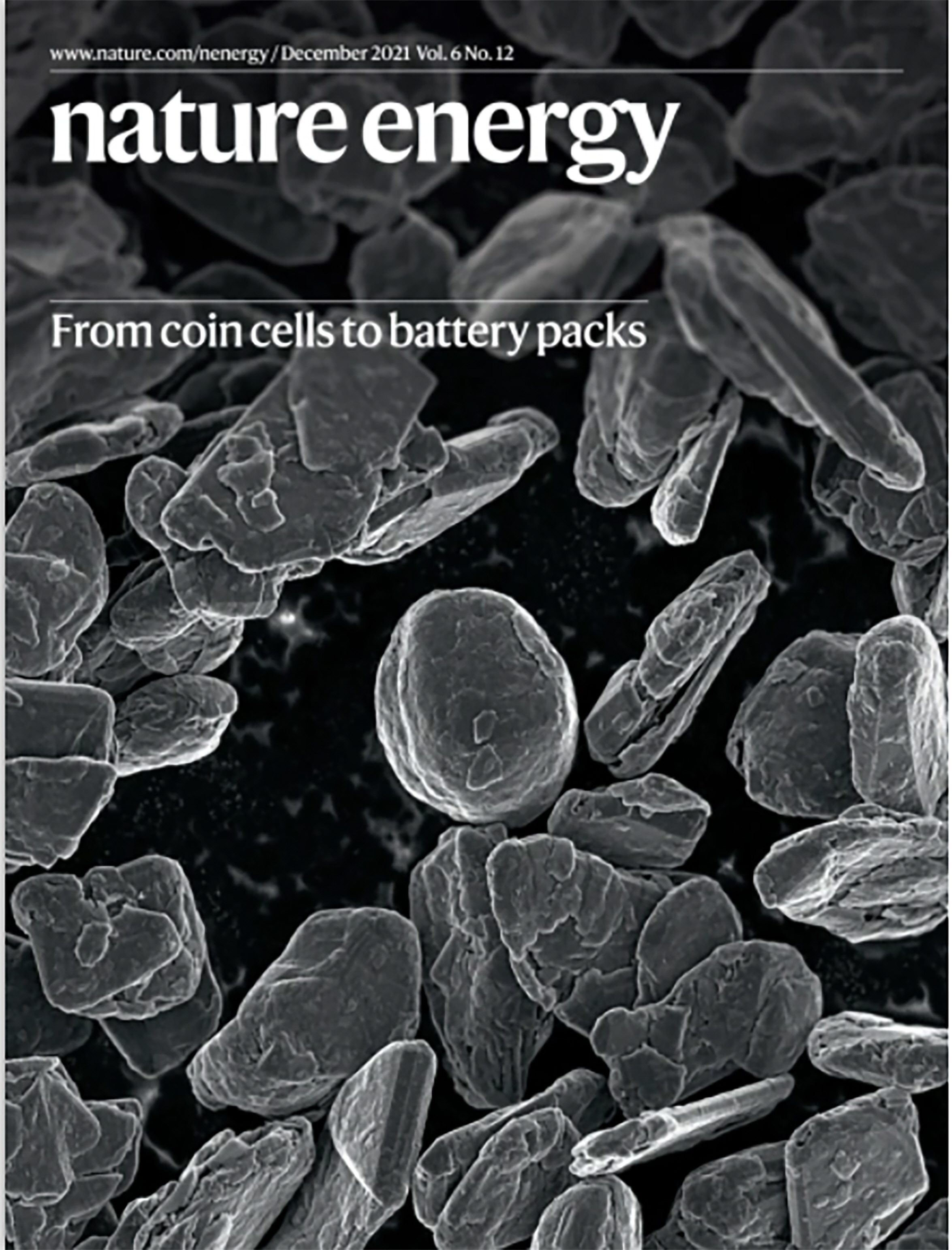
The findings of this research have been published in the December 2021 issue of Nature Energy and featured on the cover of the print edition.
The anode plays a crucial role in the high-capacity lithium-ion batteries (LIBs), as it directly influence the battery’s electrochemical performance. The more ions the anode can hold, the higher its energy capacity, and the longer the battery can run. Therefore, replacing the existing anodes with high-capacity materials is the most promising ways to achieve higher energy density Li-ion batteries, noted the research team. Silicon (Si) has been considered as a feasible candidate because of its high specific capacity. However, according to the research team, Si suffers from severe structural degradation and instability of the solid–electrolyte interphase (SEI) caused by substantial volumetric fluctuations during (de)lithiation.
Indeed, due to the large volume variation of high-capacity alloy-based anodes during cycling, it is desirable to use small anode particles for an extended battery cycle life. However, it has been challenging to realize subnano-sized particles (
Figure 1. Simulation regarding the behaviour of Si growth inhibitor. (a) Calculated heat of reaction (∆E) for the reaction of the SiH3 radical with ethylene (C2H4) and silane (SiH4). Inset: a schematic illustration of the behaviour of ethylene gas acting as a Si growth inhibitor and preventing continuous Si–Si bonding through prior bonding with Si atoms. (b,c), Schematic illustrations showing the thermal decomposition of monosilane (b) and the mixture of monosilane and ethylene (c).
In this study, the research team came up with a growth inhibition mechanism that prevents continuous enlargement of size immediately after nucleation during chemical vapour deposition. The growth inhibition is successfully applied to the synthesis of silicon, thereby yielding subnano-sized (
According to the research team, ethylene not only functions as a silicon growth inhibitor, thereby slowing the growth of nucleated silicon via Si–C bond formation, but also acts as a source to create the dual matrix. The subnano-sized silicon anode enhances the cycling stability (Coulombic efficiency reaching 99.96% over 50 cycles). Finally, the practical application of the fabricated energy storage system (103.2 kWh) containing 110 Ah full-cells with 91% capacity retention for 2,875 cycles and a calendar life of 97.6% for 1 year is demonstrated.
“These results can provide inspiration for battery researchers to consider the parameters for high-capacity alloy anode design and can pave the way for next-generation anodes in high-energy lithium-ion battery systems for application in energy storage systems and electric vehicles,” noted Distinguished Professor Cho.
Journal Reference
Jaekyung Sung, Namhyung Kim, Jiyoung Ma, et al., “Subnano-sized silicon anode via crystal growth inhibition mechanism and its application in a prototype battery pack,” Nature Energy, (2021).